Properties and functions of water
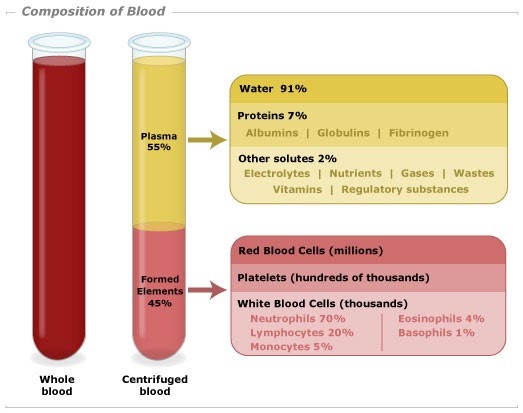
Solutes in water include sugars and proteins, as well as electrolytes such as hydrogen ions (H+), potassium ions (K+), sodium ions (Na+), chloride ions (Cl–), hydrogencarbonate ions (HCO3–), magnesium ions (Mg2+) and others.
The exact composition of different fluids varies between individuals, over time, between species, etc. In order to investigate fluid composition, various methods exist. Sugars and proteins can be detected and measured in body fluids as well as plant extracts using reagent test strips and biosensors.
For example, glucose test strips can measure the glucose concentration in a small blood sample.
Basic legacy tests for the presence of reducing sugars and proteins are the Benedict test and Biuret test. These involve adding a reagent to a test sample and observing a simple….